1 Science and the Scientific Method
Science is with us everywhere. Advances in technology and science are rapidly transforming our world – from growing food, developing medicines, making exercise regimes, recycling and presenting the daily weather report, to reading a map and using our mobile phones and computers.
The modern world would not be modern at all without the understandings and technology enabled by science. In the future, being science literate will be a necessity, not an option. We cannot escape from the significance of science.
A student scientist is someone who is being formally educated about science and how to practise science, as well as developing scientific skills and attributes such as teamwork, communication, and personal and professional responsibility.
This chapter introduces the concept of science and its importance in our lives (Sections 1.1 and 1.2). Student scientists will learn about what science is and how it works, and how science graduates are able to change society for the better (Section 1.2). Section 1.3 warns the student scientist about pseudoscience, and how it differs from ‘real’ science – what you are here to learn.
1.1 How medical science has shaped our history
Medical science includes a diverse range of specialities including anatomy and physiology, biochemistry, pathology, haematology, microbiology, immunology, and pharmacology to name a few. Within these various fields of study, many advances have been made through scientific investigation to improve our understanding of human biology, the basis of disease, and the diagnosis and treatment of disease. While the number of advancements are too vast to cover in a single text, we can gain an appreciation of the impact of these scientific advancements by a look at examples within physiology, one of the medical science areas of study.
Physiology is a branch of biology that deals with the normal functions and activities of living organisms and their parts. Physiologists are interested in the mechanical, physical and biomechanical functions of humans or animals, and their organs and cells.
Understanding and appreciating the history of physiology provides a context for learning current physiology. It also highlights how scientific understanding can change, and how current beliefs may one day be obsolete. The timeline below presents some of the notable discoveries in physiology that have informed our understanding of the human body and treatment for many diseases. These scientists have all been awarded Nobel prizes in Physiology or Medicine for their discoveries.
Box 1.1: Timeline of physiology discoveries
The website PhysiologyInfo.org, sponsored by the American Physiological Society, presents a timeline of physiology that highlights important discoveries between 1822 and 2013. General physiology milestones are presented in addition to milestones in endocrinology and metabolism.
1.2 Current issues in medical science
Every day scientific advancements that significantly enhance our understanding of physiology and quality of life are revealed. The American Physiological Society has identified six ‘hot topics’ in the life sciences that are particularly important today:
- cancer biology
- cardiovascular biology
- exercise and metabolism
- inflammation and immunity
- the microbiome (previously known as the gut flora)
- stem cells and regeneration.
Box 1.2 highlights some recent breakthroughs in four of these six key themes.
Box 1.2: Recent breakthroughs in physiology
Click on the drop-downs to read more.
1.3 Science literacy
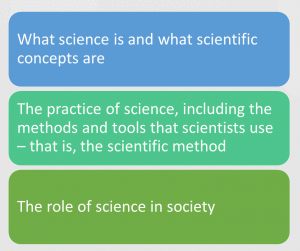
A science degree helps students develop science literacy, so that graduates can contribute to society positively. Experts including science academics, science students, employment groups and professional societies identified three elements of science literacy that a science student should understand by the time they graduate (Figure 1.1).
Graduates with scientific literacy are well prepared to participate in decision-making and debate socio-scientific issues that will improve society.
The scientific method is the procedure of systematic observation, and forming, testing and modifying hypotheses.
In high school and university, we learn science mostly from textbooks, which often presents science as absolute facts that we have always known. What the textbook doesn’t make obvious is that these facts are only the best current information we have, and that these facts are the results of years of work by many scientists experimenting.
To fully understand science, we need to know how scientists conduct science and obtain facts.
Cutting-edge knowledge at the frontier of science is not as thoroughly tested as the well-tested and long-standing knowledge existing in contemporary textbooks. New data may not stand up to the test of time. New theories, ideas and possibilities can easily disappear as research progresses and data are generated. What might have been initially imagined by the physiologist as a ‘break-through’ discovery can easily end up as nothing more than a hypothesis without data to support it.
Student scientists should have the opportunity to appreciate the full scope of science during their undergraduate degrees, which includes the freedom to imagine new ideas, but with adequate educator support to actually take on the role of a scientist and test these ideas. This way, a student can learn about and understand science.
Knowledge of science concepts, or ‘what do we know?’
The first element of scientific literacy is the most obvious – knowledge in science or the collection of facts that science has produced. Student scientists spend a large part of their undergraduate degrees learning scientific facts in their discipline area – that is, they focus on ‘what we know’ and not on ‘how we came to know it’. Scientific facts are claims about the world that can be directly established by a careful, unprejudiced use of the senses, or repeated and verifiable observations.
Scientific knowledge tells us about the nature of the world beyond what we see on the surface. It tells us about our cells and the organelles within, the transmission of energy from light hitting the retina in the eye to the electrical energy travelling down the optic nerve, and even about the conditions that existed in the world long before humans were around to observe it.
Scientific theory
The ultimate goal of science is to understand the natural world in terms of scientific theories – that is, concepts based on what we learn from experimentation. In science, our understanding is constantly increasing and, as a result, our theories develop and change.
In science, the word ‘theory’ is reserved for a conceptual scheme supported by a large number of observations; Figure 1.2 shows five currently accepted scientific theories.
Scientific theories cannot be conclusively proved or disproved, and they change when evidence requires it. Once theories are proposed to explain the facts on a particular topic, they are rigorously and ruthlessly tested by observation and experiment. Theories that don’t stand up to observational and experimental tests are eliminated and replaced by new potential explanations that must also be tested. Science progresses through the process of trial and error, by educated guesses and dismissals – only the most likely theories survive (see Box 1.3). Theories can never be described as true – rather, they are the best available explanations that are an improvement on anything we have had before.
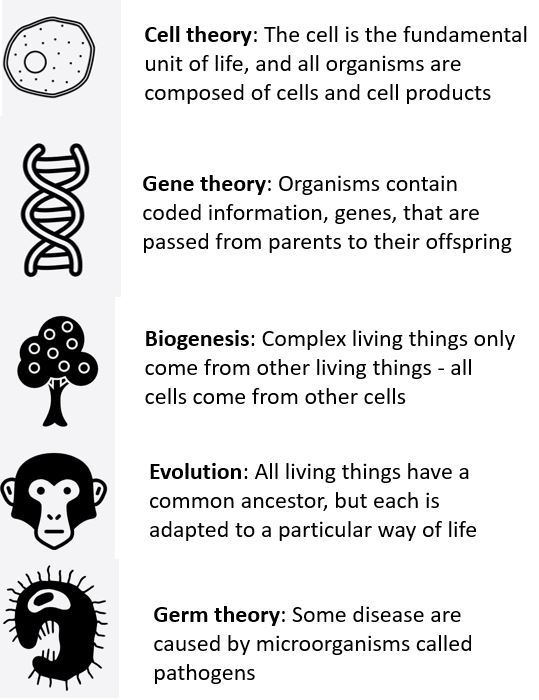
Box 1.3: Scientific hypothesis, testing and theory
Science starts with problems associated with explaining some behaviour of the world or universe in light of an existing theory. An example given by Chalmers (2013) is the observation that bats can fly well at night, even though they have small, weak eyes.
This observation is problematic in light of the theory that living creatures see with their eyes. A potential answer will be proposed to explain the observation – that is, a hypothesis. The hypothesis will then be tested and eliminated, or be successful. Once a hypothesis has managed to withstand a wide range of rigorous tests, it may become a theory and the scientist will move on to another problem and begin the process again.
It can take a lot of evidence and time to change established theories. There are many examples in science where theories were believed even when there was a great deal of opposing evidence – as explored in Box 1.4.
Box 1.4: The difficulties in changing scientific theory
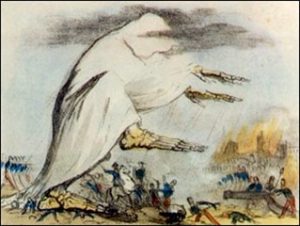
Widely accepted during the 18th and 19th centuries, the miasma theory of disease transmission explained the origin and spread of some epidemic diseases like cholera. According to the theory, these diseases were caused by miasma (Greek for pollution) – an ill-defined, poisonous vapour secreted by rotting organic matter, or a noxious form of ‘bad air’ (Figure 1.3).
In 1546, Italian physician, poet, astronomer and geologist Girolamo Fracastoro first proposed a hypothesis that contradicted the miasma theory – that contagious diseases were caused and spread by transferrable seed-like bodies. Other scientists proposed similar hypotheses , but it wasn’t until the work of Louis Pasteur and Robert Koch in the late 1860s that the germ theory became widely accepted. Joseph Lister, who came to be known as the ‘father of modern surgery’, applied the advances made by Pasteur to the hospital environment and introduced sterilisation of equipment, bedding, gowns and wounds, and washing hands to prevent infection.
Lister faced opposition despite the positive results he was achieving. It didn’t help that bacteria were too small to see – but eventually his aseptic techniques were adopted throughout Germany, the United States, France and, finally, Great Britain.
Sometimes scientists believe in a hypothesis so much that they may think they are observing things that are not there. The canals of Mars are a fascinating (although non-physiological) example of this.
In 1877, Italian astronomer Giovanni Schiaparelli reported the discovery of canals on Mars (Hetherington, 1976); many astronomers including Percival Lowell (1911) and Eugène Antioniadi (1930) also documented seeing the canals and drew detailed maps. Around 1895, these drawings led to Lowell concluding that the canals were an irrigation system developed by Martians. When more sophisticated telescopes and imaging technology were available, astronomers including Antioniadi proposed that these canals were an optical illusion caused by dust moving across the surface of Mars due to heavy winds (Gifford, 1964). This was supported by images obtained in the 1960s by unmanned NASA spacecraft.
The facts we learn about physiology as student scientists are the results of years of careful, slow work by teams of scientists. Their results – and therefore our knowledge – is constantly evolving. Textbook ‘facts’ may have been challenged, and may still be challenged and evolving.
This leads into the second element of scientific literacy: understanding how we came to know scientific facts and theories.
Knowledge of the scientific method, or ‘how did we come to know it?’
Scientific method refers to the body of techniques for investigating phenomena, acquiring new knowledge, or correcting and integrating previous knowledge. It is based on gathering observable, empirical and measurable evidence subject to specific principles of reasoning (Newton, 1726/1999, pp 794-796)
The scientific method includes the elements shown in Figure 1.4.
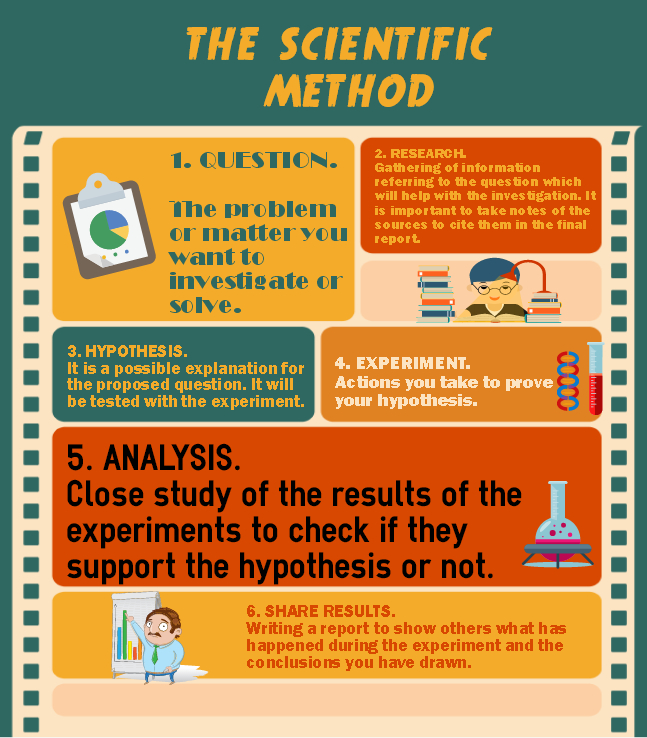
In a speech to graduating students at the California Institute of Technology in 2016, Atul Gawande, a surgeon and public health researcher, said:
Science is not a major or a career. It is a commitment to a systematic way of thinking, an allegiance to a way of building knowledge and explaining the universe through testing and factual observation (Gawande, 2016).
The process of making new discoveries in science is not as straightforward and neat as it may first appear. As a student scientist, you read textbooks, and conduct ‘cookbook’ practicals in which you follow a series of steps. In practice, creating new knowledge in science is characterised by difficulties, uncertainties and competing hypotheses
Science is a huge discipline, and the way science is done depends on the field of study. Exactly how a scientist conducts their craft depends on what knowledge and answers they are pursuing. For example, astronomers and geologists base their understanding on field observations, whereas physiologists and chemists base their understanding on experiments.
To a physiologist, the scientific method means implementing a series of experimental steps to create new knowledge and develop a more sophisticated understanding of a particular topic.
The scientific method follows seven general steps (see Figure 1.5):
- make an observation
- research what is already known about the topic (literature review)
- form a testable hypothesis that may explain the observation
- perform an experiment or series of experiments
- analyse the results
- accept or dismiss the hypothesis (conclusion)
- share the results.
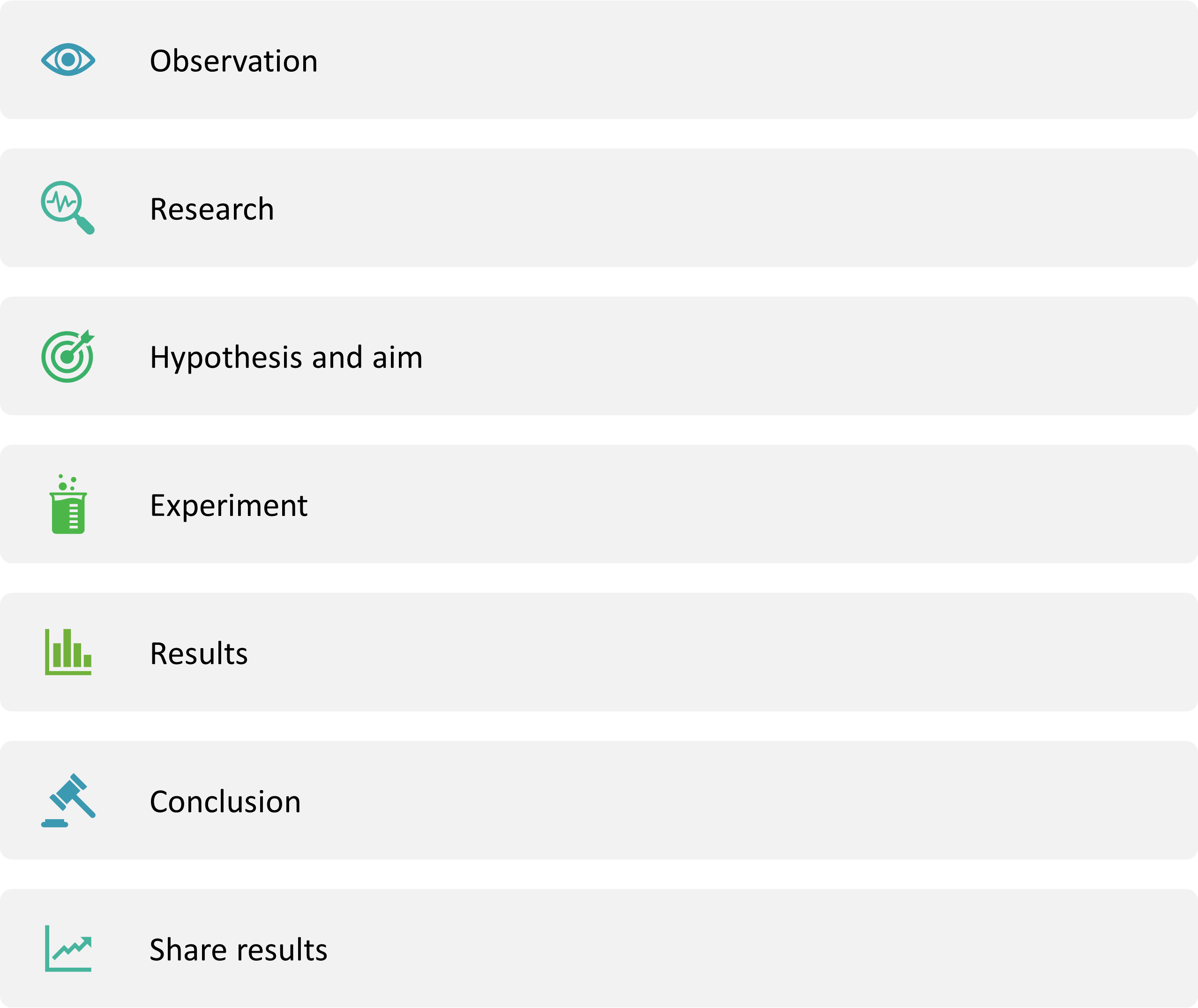
Some philosophers and scientists prefer to see the scientific method as an ideal rather than a rule or a description of the practice of all scientists. Scientists are humans, and this means that we are not perfect and we are different in terms of our motivation, drive and ideas – all of which may result in science being practised differently.
Scientists may approach their quest for new discoveries differently, but they will always seek evidence that can be obtained in different ways. The scientific method combines rational thought and imagination to predict and explain phenomena, and the work of scientists is always open to scrutiny, criticism and debate.
Figure 1.6 shows a cyclic model of the scientific method, indicating how it is an ongoing process.
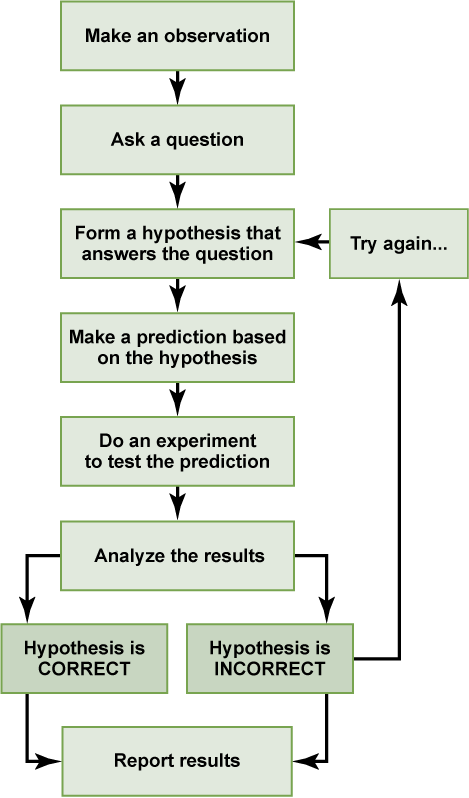
Video 1.1: Video overview of the scientific method [7 mins, 32 sec]
TED-Ed presentation by Adam Savage on two spectacular examples of profound scientific discoveries that came from simple, creative methods that anyone could have followed.
Note: Closed captions are available by selecting the CC button below.
Observation and research
Scientific research starts with a scientist making an initial observation that they cannot explain with an existing theory. It can also be a more open-ended questions, such as ‘How can I treat this particular disease?’ The scientist will study any previous data – that is, review the existing literature on the topic area, which are facts relevant to the problem at hand. This research may reveal that another scientist has already answered the question and, in this case, a new question will be asked. If the question hasn’t been answered, the scientist will use the findings of their research to help them to design a good question that they can test.
Hypothesis and aim
Research, and imaginative and creative thinking help the scientist to formulate a hypothesis that they can test to explain an observation or answer a question. A hypothesis is an assumption based on knowledge obtained during the observation stage – it is an ‘educated guess’.
Data generated during an experiment either support or fail to support the hypotheses. Hypotheses may be specific (e.g. chronic ingestion of artificial sweeteners causes weight gain in humans) or broad (e.g. viruses cause disease in animals). Hypotheses are always subject to modification.
It is important to note that hypotheses, however, may be proven untrue. This occurs when the data do not support the hypothesis, in which case, the hypothesis must be rejected or refined.
A hypothesis is tightly linked to the aim of the study, which is the objective or goal of an experiment or series of experiments. The aim is written in a similar manner to the hypothesis. A hypothesis is a prediction about the outcome of an experiment; an aim is usually written in the form of an instruction:
Hypothesis: chronic ingestion of artificial sweetener causes weight gain in humans.
Aim: To determine if chronic ingestion of artificial sweetener causes weight gain in humans.
Experiment
Physiologists conduct experiments to test whether the real world behaves as predicted by the hypothesis. The purpose of the experiments is to find out if the resulting observations of the real world agree or conflict with the prediction – if they agree, we can be more confident that the hypothesis is true, but if they disagree we become less confident.
Experiments are a valuable scientific tool, because when experimenting we are able to control and manipulate phenomena and events. However, this also means that we are creating an ‘artificial environment’ for our experiments. This may not end up reflecting what is happening in the real – messy – world.
Experiments will only help us to support or not support hypothesis if they are carried out properly. This means using care and the right equipment and settings, and the with goal of minimising error. If we conduct careless experiments that introduce error into the results, the observations (or data) are useless when we try to evaluate our hypothesis.
Part of understanding how science works includes becoming aware of the methods and tools of science. Each scientific discipline has its own specialised techniques and tools that it uses to observe the natural world; student scientists will be exposed to some of these methods in practical classes during their studies. Experimental methods are not perfect, even when scientists try their best, and they can be updated or replaced over time. Advances in technology or scientific understanding may mean that previously accepted methods are rejected and replaced with new ones. This may also mean that experimental results obtained using old methods are also ignored, or viewed as less relevant, because our way of thinking about the topic has changed.
It is not always easy and straightforward to conduct well-designed experiments and to get reliable results. New experiments may take months or even years to perfect. Scientists need to work hard to collect high-quality results, including:
- developing their theoretical and practical knowledge of an area and a technique
- learning through trial and error
- mastering the available technology.
Researchers must provide details on how their experiments were conducted, because other researchers must be able to repeat the results. If results cannot be repeated, the hypothesis is no longer supported, and the conclusion may be incorrect. When the results of experiments are shared with the wider scientific community, the research will be judged on many things, including the methods employed.
Also, it is very rare for a researcher to generate their experimental methods from scratch; it is more common to use methods that have been shown by other researchers to be successful at measuring particular variables. This is another reason it is important to provide details of your methodology.
Results
Scientists collect the data generated from their experiments and analyse them to determine whether the hypothesis was supported or not. In the analysis stage, the scientist combines the results from repeated experiments and carries out statistical analysis to test the hypothesis – for example, to determine whether differences exist between different experimental groups. Scientists will present their results as text, tables and graphs.
Results should be reproducible – that is, another scientist should be able to follow the same method and obtain the same results. If they are not able to do this, it may mean that the original results were an error. For this reason, scientists will usually conduct a single experiment multiple times.
Scientists rarely attempt to replicate the findings of other scientists, despite the importance of reproducibility. There are few incentives for scientists to dedicate time to replicating existing studies, and it can be difficult to replicate studies because the methods may be unclear, and poor or incorrect study designs may have been used in the original research (Belluz et al., 2016).
Recently, some scientists have found that when they do try to replicate studies, they have not been able to obtain the same results. This has led to a ‘crisis of reproducibility’. Scientists who have investigated reproducibility have found that few findings published in top psychology journals as well as highly cited papers on psychiatric treatment (Tajika et al., 2015) could be replicated (Open Science Collaboration, 2015). This issue is gaining more attention and must be addressed by scientists from all disciplines.
Conclusion
Conclusions are made about the results of the experiment in light of the hypothesis. Simply, the results do or do not support the hypothesis. The conclusions below are the two possibilities arising from the example hypothesis and aim shown previously:
Conclusion: Chronic ingestion of artificial sweetener caused weight gain in humans.
Conclusion: Chronic ingestion of artificial sweetener did not cause weight gain in humans.
The study hypothesis, aim and conclusion are therefore highly connected.
Scientific conclusions are subject to change when new findings dictate that they need to be changed. Quite often in science, new studies, which might use new techniques and equipment, tell us when conclusions need to be modified or changed entirely.
Share results
Results of experiments should be shared with the wider scientific community, so they can contribute to the pool of knowledge on a topic and lead to better understanding for everyone.
Scientists can communicate with their peers in different ways:
- make an oral presentation at a conference
- present a poster at a conference
- publish a journal article in a peer-reviewed academic journal (see Box 1.5).
Publication of results makes information widely available and the results will be used by others if they are seen as interesting – results not deemed interesting will fade from sight; sadly, this is the fate of most published articles.
Box 1.5: Peer-reviewed articles and academic journals
Results do not become a part of the pool of shared knowledge until they are published, but not all results achieve this outcome. Scientists must present their findings in the accepted format – that is, a journal article, and convince journal editors and referees that their work is interesting, sound and useful.
Once a journal editor accepts an article, it is subjected to peer review. This is where others scientists with expertise in the relevant field, usually anonymously, read through and check that the work is of sufficient quality and worthy of publication. The publication and peer-review process is at the mercy of people and therefore not perfect – error, bias and dishonesty is inherent. As a result, some research that should be published isn’t – and some research that shouldn’t be published is.
This is a stressful process because research scientists in universities and industry aim to publish their work in academic and professional journals. Their publication record is tremendously important to scientists because it is often used to judge the quality of their work. Ultimately, a researcher’s publication record determines whether they get or keep jobs, or gain funding to continue their work.
Interesting and important results will be cited by other scientists in the field who will test the results, and often modify and extend on the research. They may also report back if they find something wrong or inadequate in the research. Results that withstand scrutiny by peers and continue to be seen as interesting, useful and correct (or not obviously wrong) will make their way into secondary literature, that is review articles, monographs and graduate-level textbooks. If more time passes and the results are still interesting and correct, they will find their way into undergraduate textbooks, which have the widest reach and influence.
An example of the scientific method is shown in Box 1.6.
The language used in presentations and journal articles is highly technical and will contain a lot of scientific jargon. A non-expert audience will struggle to understand the meaning of such publications. To communicate their findings to lay – or non-expert – audiences, scientists may talk about their work during, for example, a radio or television interview.
Chapter 6 and Chapter 7 discusses the ins and outs of writing technical publications, and Chapter 8 talks more about communicating science to the non-expert audience.
Box 1.6: The scientific method in action
Observation: A physiologist determines that available evidence indicates that say drug X – which is not yet approved by Australia’s Therapeutic Goods Administration – increases the metabolic rate, and may therefore be a promising weight loss drug.
Hypothesis: The physiologist hypothesises that ‘drug X will cause weight loss in adult rats’.
Aim: The physiologist plans a study, with the aim to ‘determine if drug X will cause weight loss in adult rats’.
Experiment: The physiologist randomly assigns 20 adult rats to a control and treatment group – 10 per group. The control group is administered a daily dose of the placebo and the treatment group is administered a daily dose of the drug for 4 months. The control group receives a placebo instead of a drug, and all other conditions are identical to the treatment group with the exception of the drug. They receive the same kind of food and water. Body weight is recorded daily.
Results: At the end of the 4-month treatment period, the physiologist performs a statistical analysis on the body weight data. The data reveal that there is a statistical difference between treatment groups, with the treatment rats weighing less than the control rats.
Conclusion: Drug X caused weight loss in adult rats.
Share results: The physiologist will share their work with peers through a poster or oral presentation at a conference, and/or a journal article.
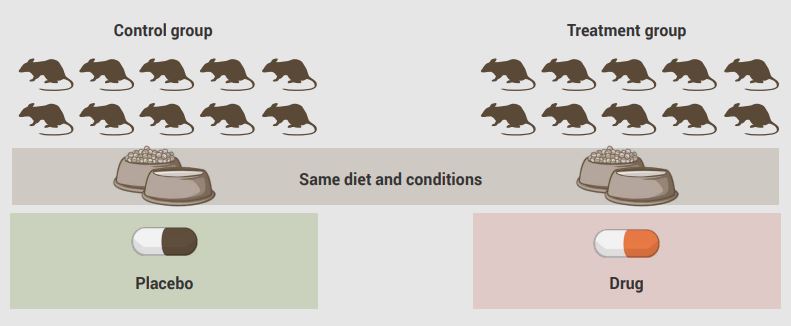
Knowledge and appreciation of science in society, or ‘how can we contribute’?
Understanding how science influences society – and vice versa – is an important aspect of scientific literacy. Science graduates who are able to combine their scientific knowledge and how it works with an understanding of the role of science in society will be valuable citizens who can contribute effectively to debates and decision making about science-related public issues.
One of the roles of a science graduate is to uphold the reputation of science in society by explaining what it is and why it is important. Many members of the public misunderstand what science is, what it means if something is scientific, and how science and technology are related. Some scientists may also share these misunderstandings (Bauer, 1992).
Video 1.2: Science and Society [4 mins, 37 secs]
Watch this video interview with Steven Pinker, renowned experimental psychologist and Professor in the Department of Psychology at Harvard University, on the place of science in society.
Note: Closed captions are available by selecting the CC button below,
Many people already do respect science, and this has been exploited in some advertisements that tell us that a product has been ‘scientifically proven’ to be better, stronger, faster, more efficient or even sexier than its rivals. This is meant to make the product more attractive to consumers because it implies that the claims are well founded and perhaps beyond dispute (Chalmers, 2013).
On the other hand, some people do mistrust science, as evidenced by some views about climate change and childhood vaccinations. Although most scientists agree that climate change is occurring, some of the public still view it as an issue that is up for debate. And despite the evidence supporting the positive impact of immunisation on human health, some parents are reluctant to vaccinate their children.
Scientists are responsible for discoveries that have resulted in nuclear weapons and pollution, and – as a result – are distrusted by some members of the public. A study published in 2012 reviewed data collected from American citizens on their social attitudes from 1974 to 2010 (Gauchat, 2012). The author found that trust in science has not declined during this time period, except among people who described themselves as politically conservative and those who frequently attended church. Also, levels of trust in science varied a lot according to social class, ethnicity, gender, and region. This is not surprising, given the way science and scientists have treated some populations – for example, African–American men in the Tuskegee study (see Case Study 1.1).
Case Study 1.1: Tuskegee study and distrust of doctors among African–Americans
Marcella Alsan and Marianne Wanamaker published a working paper in the National Bureau of Economic Research in 2016 (Alsan & Wanamaker, 2016); the abstract summarises their study.
Alsan and Wanamaker’s research showed that the unethical study may have led to a distrust in doctors, which contributed to (~35%) African–American men avoiding the health care system and therefore dying earlier. This effect was stronger the closer the men lived to Macon County, Alabama, where the Tuskegee study took place.
Although science influences how society functions, it is also true that society influences which topics are chosen for research and government funding. In 2015, the Australian Government developed a set of Science and Research Priorities. This project was led by the former Chief Scientist, Professor Ian Chubb, and included consultation with researchers, industry leaders and government representatives, who together represent all citizens. The areas that have been chosen as priorities are those deemed to be of critical importance to Australia and will be given public funding. They are:
- Food
- Soil and water
- Transport
- Cybersecurity
- Energy
- Resources
- Advanced manufacturing
- Environmental change
- Health
1.4 Pseudoscience
“Pseudoscience is the form of science without the substance.” (Gawande, 2016)
Pseudoscience describes an idea, field, practice, body of knowledge or belief that is presented as being scientific, but does not follow or obey the scientific method, and is not consistent with the standards of scientific research.
In 2006, the National Science Foundation (in the United States) analysed survey data from the previous 20 years and found that many Americans have many pseudoscientific beliefs, including astrology, lucky numbers, the existence of unidentified flying objects (UFOs), extrasensory perception (ESP) and magnetic therapy (National Center for Science and Engineering Statistics, 2006). They found that belief in pseudoscience increased during the 1990s and early 2000s, but declined between 2001 and 2005. In 2012 (National Center for Science and Engineering Statistics, 2014):
- 55% of Americans said astrology is ‘not at all scientific’
- 32% said they thought astrology was ‘sort of scientific’
- 10% said it was ‘very scientific’
- about 4% said they ‘did not know’.
These numbers have not changed dramatically since surveys began, indicating that there is much for science communicators to achieve. Other examples of pseudoscience include acupuncture, alchemy, astrology, homeopathy, intelligent design and physiognomy.
Box 1.7: Characteristics of pseudoscience
- The use of vague, exaggerated, or untestable claims: Many claims made by pseudoscience cannot be tested with evidence. As a result, they cannot be falsified, even if they are not true.
- An over-reliance on confirmation rather than refutation: Any incident that appears to justify a pseudoscience claim is treated as proof of the claim. Claims are assumed true until proven otherwise, and the burden of disproof is placed on skeptics of the claim.
- A lack of openness to testing by other experts: Practitioners of pseudoscience avoid subjecting their ideas to peer review. They may refuse to share their data and justify the need for secrecy with claims of proprietary or privacy.
- An absence of progress in advancing knowledge: In pseudoscience, ideas are not subjected to repeated testing followed by rejection or refinement, as hypotheses are in true science. Ideas in pseudoscience may remain unchanged for hundreds — or even thousands — of years. In fact, the older an idea is, the more it tends to be trusted in pseudoscience.
- Personalization of issues: Proponents of pseudoscience adopt beliefs that have little or no rational basis, so they may try to confirm their beliefs by treating critics as enemies. Instead of arguing to support their own beliefs, they attack the motives and character of their critics.
- The use of misleading language: Followers of pseudoscience may use scientific-sounding terms to make their ideas sound more convincing. For example, they may use the formal name dihydrogen monoxide to refer to plain old water (Miller, 2020).
Defenders of science have identified hallmark moves of the pseudoscientist, as shown in Box 1.7.
Scientifically literate graduates are able to improve society by combatting pseudoscience, as discussed in the section ‘Knowledge and appreciation of science in society’.
Resource
Australian researchers John Cook and Professor Stephan Lewandowsky wrote The Debunking Handbook, a guide to defending science as a more valid approach to explaining the world.
Click the drop down below to review the terms learned from this chapter.
Copyright note: Content from the following source is reproduced with permission from the copyright holder and is excluded from the Creative Commons Licence of this work. No further reproduction of this quotation is permitted without prior permission from the copyright holder.
- Gawande, A. (2016). The mistrust of science. The New Yorker.
Additionally, content from:
- Miller, C. (2020). Human Biology. Thompson Rivers University is used under a CC-BY-NC 4.0 licence.
- Australian Government Science and Research Priorities is used under a CC-BY 2.0 licence.
References
Alsan, M., & Wanamaker, M. (2016). Tuskegee and the health of black men. (Working Paper 22323.). National Bureau of Economic Research. https://www.nber.org/system/files/working_papers/w22323/w22323.pdf
Bauer, H.H. (1992). Scientific literacy and the myth of the scientific method. University of Illinois Press.
Belluz, J., Plumer, B., & Resnick, B. (2016, September 7). The 7 biggest problems facing science, according to 270 scientists. Vox. https://www.vox.com/2016/7/14/12016710/science-challeges-research-funding-peer-review-process
Chalmers, A.F. (2013). What is this thing called science? (4th ed.). Hackett Publishing.
Gauchat, G. (2012). Politicization of science in the public sphere: A study of public trust in the United States, 1974 to 2010. American Sociological Review, 77(2), 167-187
Gawande, A. (2016, June 10). The mistrust of science. The New Yorker. https://www.newyorker.com/news/news-desk/the-mistrust-of-science
Gifford, F.A. (1964). The Martian canals according to a purely aeolian hypothesis. Icarus, 3(2), 130-135. https://www.sciencedirect.com/science/article/abs/pii/0019103564900545
Haynes, R.D. (1994). From Faust to Strangelove: Representations of the scientist in western literature. John Hopkins University Press.
Hetherington, N.S. (1976). The British Astronomical Association and the controversy over canals on Mars. Journal of the British Astronomical Association, 86, 303-308. https://articles.adsabs.harvard.edu//full/1976JBAA…86..303H/0000303.000.html
Miller, C. (2020). Human Biology. Thompson Rivers University. https://humanbiology.pressbooks.tru.ca/
National Center for Science and Engineering Statistics. (2006). Chapter 7: Science and technology: Public attitudes and understanding. Retrieved July 22, 2016, from http://wayback.archive-it.org/5902/20150818094952/http://www.nsf.gov/statistics/seind06/c7/c7s2.htm
National Center for Science and Engineering Statistics. (2014). Chapter 7: Science and technology: Public attitudes and understanding. Retrieved July 22, 2016, from https://www.nsf.gov/statistics/seind14/content/chapter-7/chapter-7.pdf
Newton, I. (1999). Philosophiae Naturalis Principia Mathematica, (3rd ed.). (I. B. Cohen & A. Whitman 1999, Trans). University of California Press. https://archive.org/details/principiamathema0000newt/page/n9/mode/2up (Original work published in 1726).
Open Science Collaboration. (2015). Estimating the reproducibility of psychological science. Science, 349(6251), Aug 28.
Pitt, D., & Aubin, J.-M. (2012). Joseph Lister: Father of modern surgery. Canadian Journal of Surgery. 55(5), E8-E9. https://doi.org/10.1503/cjs.007112
Tajika, A., Ogawa, Y., Takeshima, N., Hayasaka, Y., & Furukawa, T.A. (2015). Replication and contradiction of highly cited research papers in psychiatry: 10-year follow-up. British Journal of Psychiatry, 207(4), 357-62. https://doi.org/10.1192/bjp.bp.113.143701
Yucel, R. (2013). Good practice guide (science): Threshold learning outcome 1 Understanding science. Australian Government Office for Learning and Teaching. https://www.acds.edu.au/wp-content/uploads/Science-Good-Practice-Guide-2013-TLO1.pdf